By Sarah Derouin, Ph.D.
If you’ve ever stood on a wave-soaked beach, you’ve probably experienced small-scale liquefaction. Maybe your feet gradually sank in the sand under your own weight (known as static or flow liquefaction), or you wiggled your toes, creating mini seismic waves that shook the wet sand, slowly sending you deeper as the ground went from solid to liquid (known as cyclic liquefaction). In both scenarios you were standing on a compactable material that had a lot of water dispersed between the grains of sand.
Zooming out from the shoreline to the built environment, seismic waves from earthquakes can trigger soils under buildings and roadways to lose their strength — that is, liquefy — and behave like fluids. The results can be profound — buildings can tilt and sink, underground infrastructure like pipelines can float to the surface, roads can crack and crumble, and people can be harmed or killed in the aftermath.
Traditionally, liquefaction was considered a potential problem when there were undrained soils and a triggering event, like high-energy earthquakes. But there have been many earthquake-liquefaction events that don’t fit into this neat scenario — some soils liquefy far from the earthquake epicenter under low-energy shaking conditions in far-field locations.
Far-field locations are considered places at distances greater than one fault rupture length from the hypocenter of an earthquake — a spot where the seismic energy is well below the 30 joules per sq m threshold needed to trigger near-field liquefaction.
So what are the driving forces in these locations?
In “Drainage explains soil liquefaction beyond the earthquake near-field,” a paper published Sept. 27 in Nature, researchers reported on their investigation into how soils can liquefy in drained conditions using laboratory experiments. They found that in drained conditions there is still enough fluid in the soils for liquefaction to occur, even under low-seismic, far-field conditions. They note their work highlights the importance of considering liquefaction hazards for a variety of environments.
Seismic-induced liquefaction
Liquefaction is an expected hazard that occurs in relatively close proximity to the epicenter of earthquakes in wet areas that experience intense shaking. In 1989, the Loma Prieta earthquake that had its epicenter about 97 km from San Francisco liquified sandy materials that had been used to fill in a lagoon in 1915 to create the Marina District within the city.
But there have been many documented cases of liquefaction in far-field areas — away from the highest-energy seismic waves, explained Liran Goren, Ph.D, an associate professor of geology at Ben-Gurion University of the Negev and co-author of the Nature article, who answered questions for Civil Engineering Online on behalf of all the authors. “Many of the liquefaction events that occurred during the famous Canterbury 2010-2011 earthquake sequence (that caused an enormous amount of damage in Christchurch, New Zealand) occurred in the far field, under very low-seismic energy density input,” the researchers say via Goren.
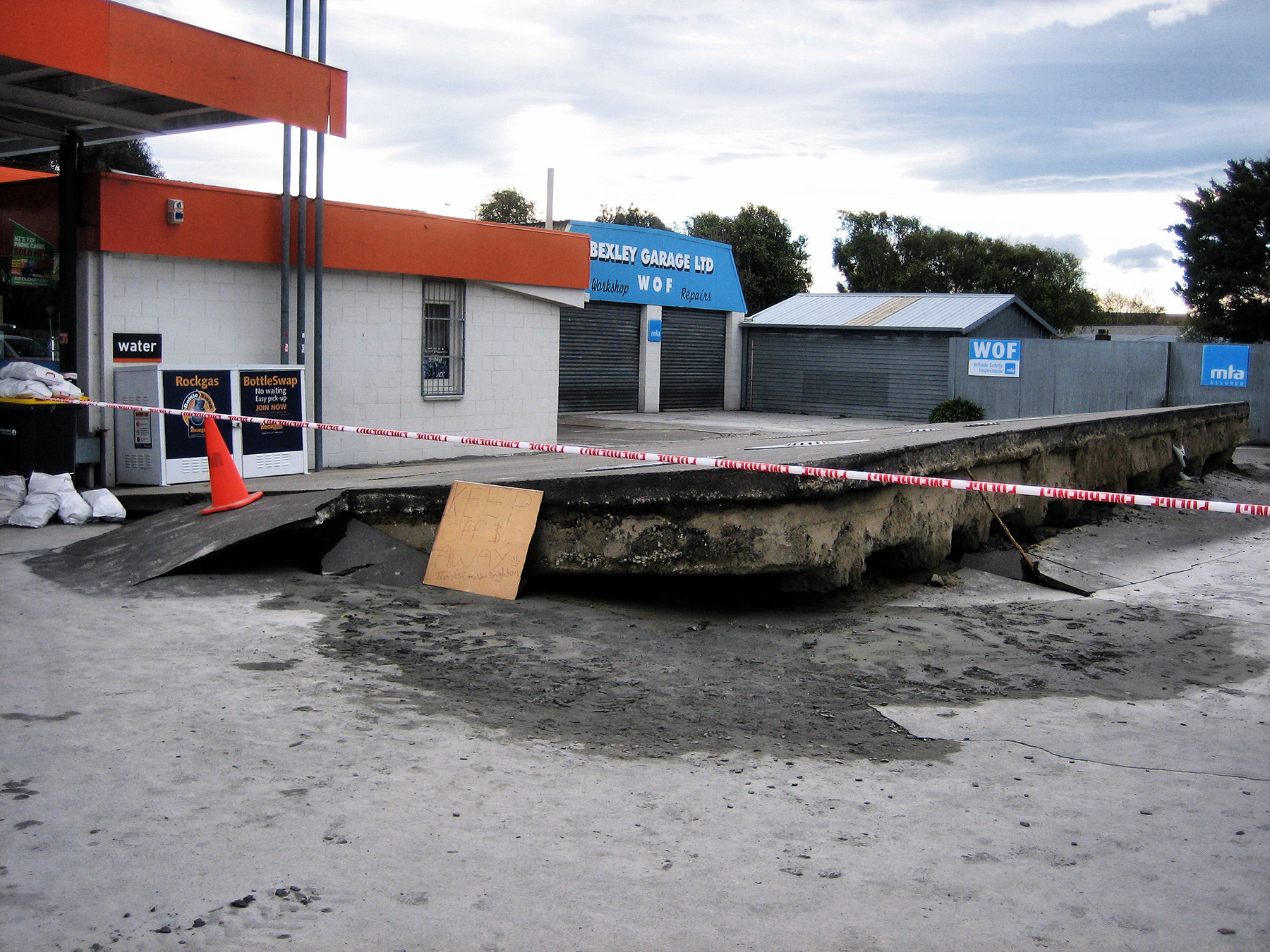
The 2010 Canterbury earthquake was a moment magnitude 7.1 event that had its epicenter 40 km west of Christchurch. Aftershocks continued through 2011.
The authors of the study also include Shahar Ben-Zeev, Ph.D., postdoctoral researcher in geophysics at the Hebrew University of Jerusalem; Renaud Toussaint, Ph.D., CNRS, the director of research at the National Center for Scientific Research at the Earth and Environment Institute of Strasbourg; and Einat Aharonov, Ph.D., professor of geophysics at the Hebrew University of Jerusalem.
Intrigued by these and other liquefaction events around the world, the researchers “wondered if drained liquefaction could be invoked to explain those puzzling, far-field events,” the researchers say.
To test this, the team created a transparent tank filled with saturated silt grains. Team members induced shaking, filmed the grain motion with a high-speed camera, and measured fluid pressures. “By performing experiments and simulations, we could establish correlations between local strain within the grain layer and the flow and pressurization of the pore fluid,” they say. “This approach provided valuable insights into the interplay between granular materials and fluid dynamics.”
Lastly, the team developed a physics-based theory to predict the preferred conditions for liquefaction in drained soils. (While undrained soils include a combination of trapped water and soil, drained soils are either dry or have clear pathways for water to escape during shaking events.) The researchers discovered that low levels of seismic shaking can still trigger interstitial fluid flow in drained conditions.
Based on observations in the lab, the team proposes that there is a “mechanistic link between the effective drainage conditions we applied in the experiments and simulations and the possibility of liquefaction with small seismic energy density.”
Liquefaction and drained soils
When earthquake waves pass through undrained soil, the water counteracts the soil’s ability to compact and causes the sediment to lose any resistance to shear forces, resulting in liquefaction. With drained soils, however, as the grains become more compacted, the small amount of water that is still present within the soil moves toward the surface.
This process can cause a surprising effect. The researchers found that during an earthquake, the seismic waves create a compaction front moving upward through the ground in far-field locations. This wave essentially divides the soil column into a compacted layer at the bottom and a liquefied layer at the top as the water is pushed out. They pointed to a video shot shortly after the 2011 Tohoku, Japan, earthquake, showing how the water escapes to the surface.
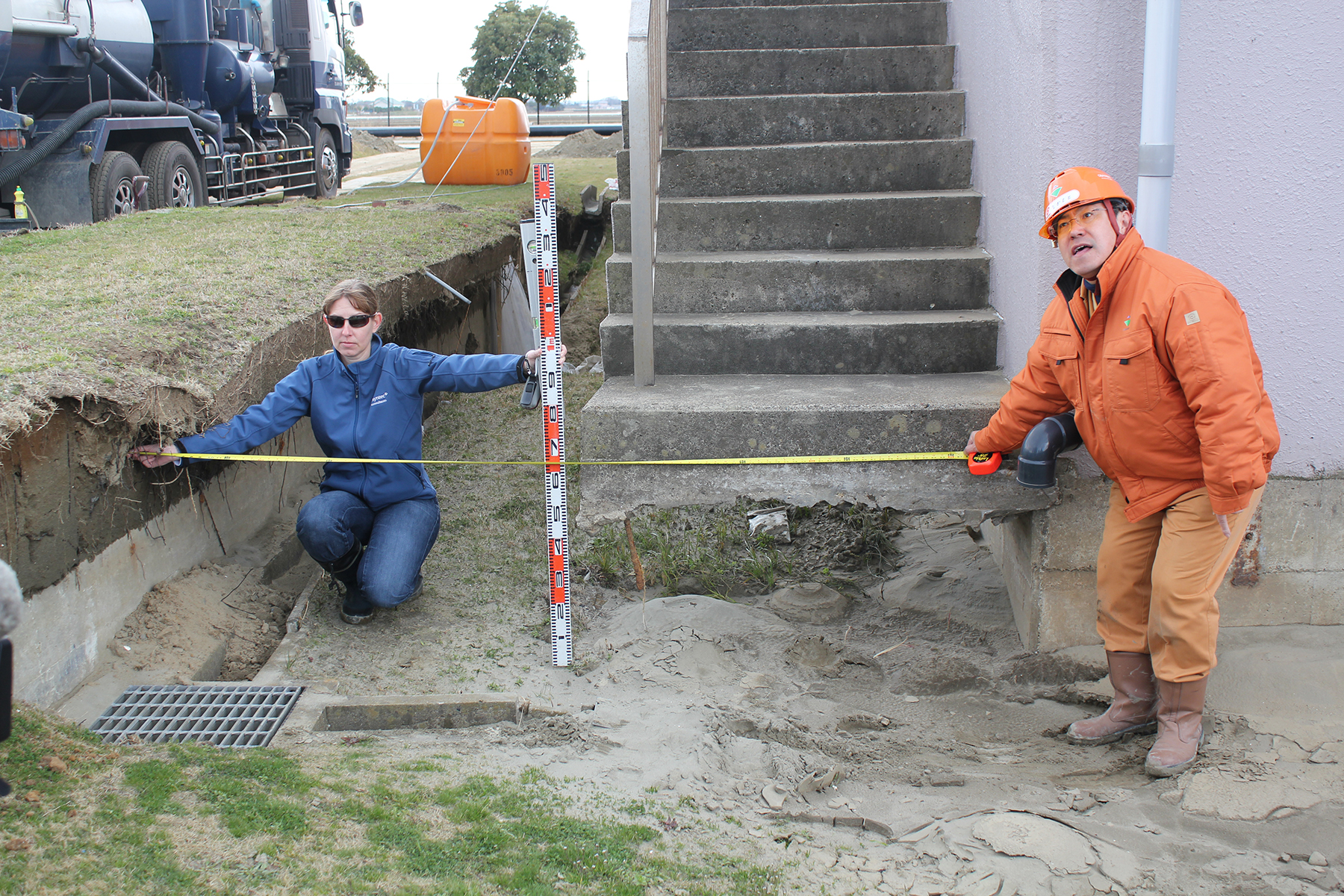
“Our research reveals that drained liquefaction is triggered by the fluid flow itself. As the soil compacts due to deformation and disturbance of contacts between grains, the fluid begins to move upward and out of the compacting soil, and pore pressure gradients build up to support the draining pore fluid,” they explain.
What’s more, the team said that the key factor influencing whether drained liquefaction occurs is seismic power or the magnitude and duration of shaking. “This intensity property is rarely calculated or discussed, and we think it is worthwhile exploring the correlation between the seismic power and the occurrence of liquefaction using natural liquefaction catalogs,” they add.
“Like in many other scientific projects, what we discovered at the end is not exactly what we hunted for in the beginning,” the researchers note. “We didn’t start with the hypothesis that drained liquefaction can be triggered with a very low-seismic energy density input. This discovery emerged from the simulations, experiments, and theory.”
While the results are a “step forward” in liquefaction research, Jorge Macedo, Ph.D., P.E., A.M.ASCE, a civil engineering assistant professor at Georgia Institute of Technology, pointed out the experiments took place in idealized laboratory conditions. “We know that liquefaction in the field is not going to be either fully drained or undrained; it’s going to be partially drained.”
The team members are positive about their findings, however, noting that “this newfound insight could shed light on the mechanisms behind numerous liquefaction events happening far from the earthquake's epicenter, where seismic energy density is low — an aspect that was previously considered a mystery.”
This article is published by Civil Engineering Online.