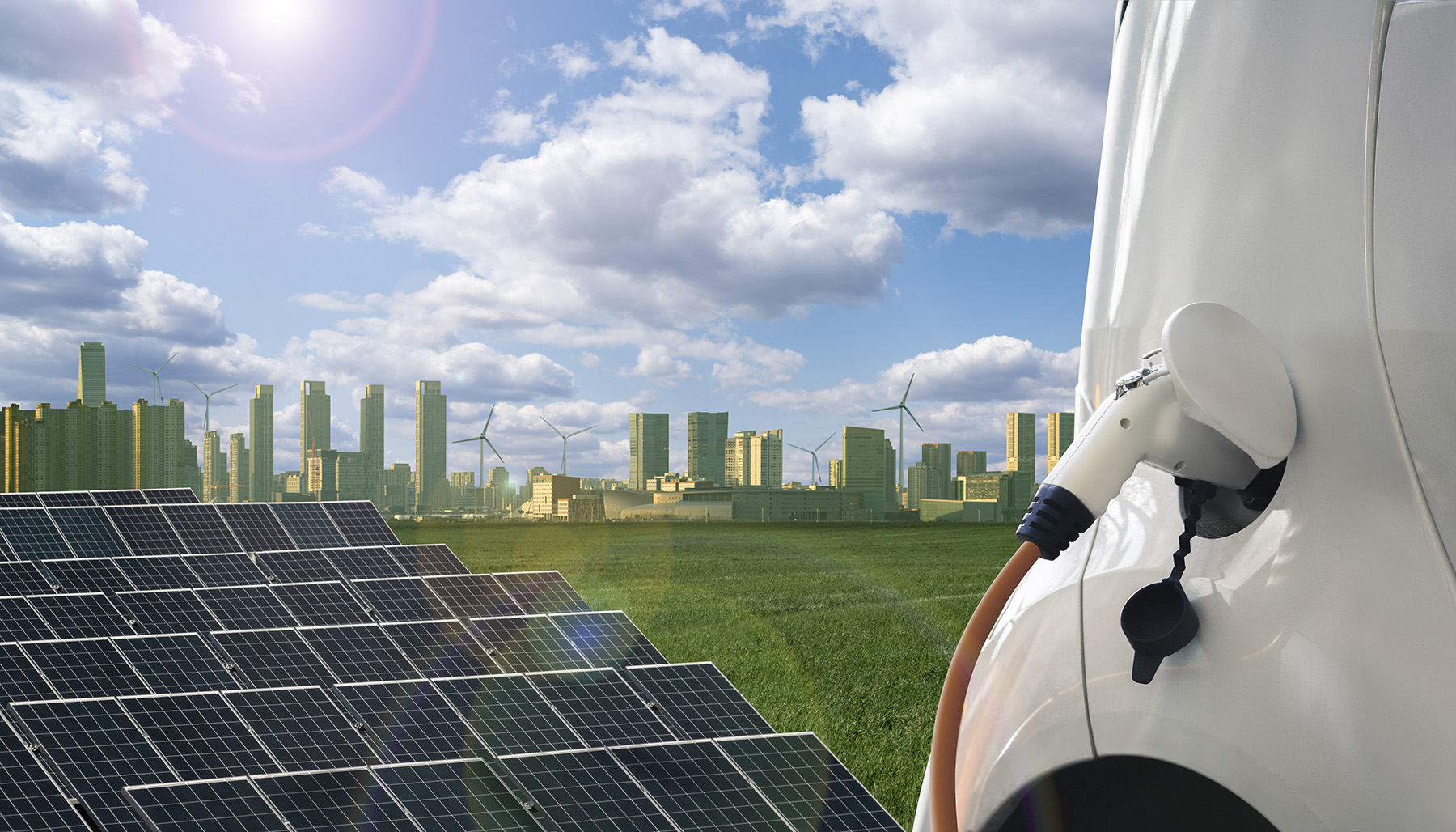
By Robert L. Reid
Because cities are a major source of greenhouse gas emissions, structural and other civil engineers are working to decarbonize buildings, power generation, transportation systems, and other urban infrastructure.
“Cities are major contributors to climate change,” consuming 78% of the world’s energy and producing more than 60% of global greenhouse gas emissions, explains a United Nations website about cities, pollution, and climate change solutions.
A key factor in this metropolitan problem is that most of the electricity that powers cities is still generated by burning fossil fuels: Only about 29% of the world’s electricity currently comes from renewable sources, such as wind and solar, the U.N. notes. But “cleaner sources of energy are gaining ground,” and the cost of renewable energy technologies “also keeps falling, making renewables the most affordable source of power today,” the U.N. states.
To help offset the impact that urban areas have on climate change, cities large and small across the United States have launched efforts at decarbonization that range from broad “green new deal” programs to more focused efforts, such as discouraging the construction of new glass and steel skyscrapers — as New York City leaders proposed in 2019 — and requiring that all new buildings be heated by electricity, banning new installations of natural gas in houses, apartments, or commercial buildings, as Berkeley, California, did that same year.
Various cities are working to electrify their fleets of buses or other vehicles, and hundreds of cities across the nation have joined a cooperative organization to combine their purchasing power for electric vehicles and charging infrastructure.
Chicago recently adopted an energy rating system for buildings of 50,000 sq ft or larger, the results of which must be publicly displayed in a prominent location, and New York has passed a measure known as Local Law 97 that fines the owners of certain buildings if they do not reach specific emission reduction goals. Boston has a similar law known as BERDO 2.0, which refers to the second phase of the Building Emissions Reduction and Disclosure Ordinance.
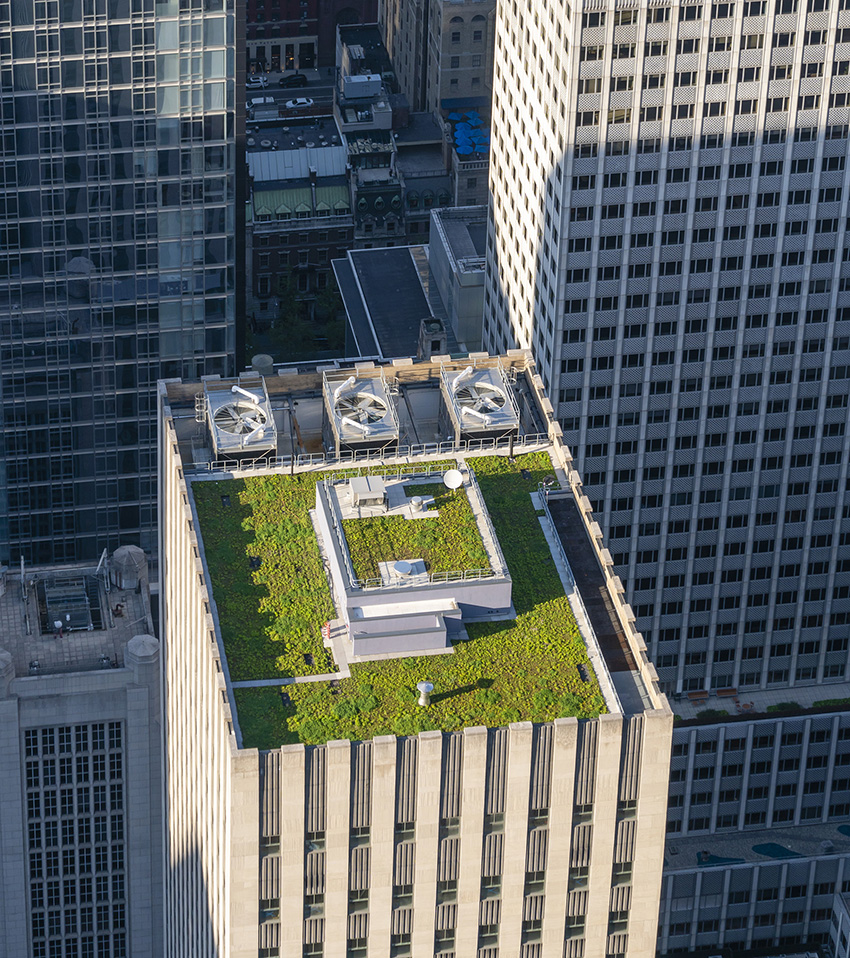
San Francisco has committed itself to powering its downtown with 100% renewable energy by 2030. As part of an effort to be carbon neutral by 2050, Boston developed a study, Carbon Free Boston, that focused on citywide emissions and measures to achieve carbon neutrality. The city also prepared a set of guidelines promoting zero-emissions buildings to reduce energy use and eliminate carbon emissions in affordable housing projects.
Los Angeles, New York, Denver, and Portland, Oregon, have also launched efforts that require new buildings to be topped by green roofs, so-called cool roofs, solar panels, and/or wind turbines — all of which are, at least in part, aimed at reducing the urban heat island effect, cutting energy demand, and otherwise combating the effects of climate change.
Civil engineers are working with urban leaders to decarbonize their energy grids, buildings, transportation systems, and other sources of emissions. They are also taking into account the embodied carbon of those urban infrastructure systems, which is a combination of the emissions generated when the structures and other systems were originally built as well as the emissions generated when the materials used in those systems were transported, produced, processed, or even dug out of the ground.
Finding new power
The need to transition energy systems from fossil fuels to zero-emission sources is “critical to mitigating climate change, protecting human health, and revitalizing the U.S. economy,” according to a February 2021 report, Accelerating Decarbonization of the U.S. Energy System, produced by the National Academies of Sciences, Engineering, and Medicine.
Such a transition is “technologically feasible” but will require fundamental shifts in how the nation generates, stores, uses, and invests in energy, notes Chris Hendrickson, Ph.D., NAE, Dist.M.ASCE, the Hamerschlag University Professor Emeritus in the department of civil and environmental engineering at Carnegie Mellon University. Hendrickson served on the National Academies’ Committee on Accelerating Decarbonization in the United States: Technology, Policy, and Societal Dimensions, which prepared the Accelerating Decarbonization report.
For example, a summary document highlighting the key points of the report noted that the goal of converting 75% of the nation’s electrical generation to renewable sources by 2030 will require “record-setting deployment of solar and wind technologies” as well as scaling back coal and some gas-fired power plants while also preserving existing nuclear and hydroelectric facilities.
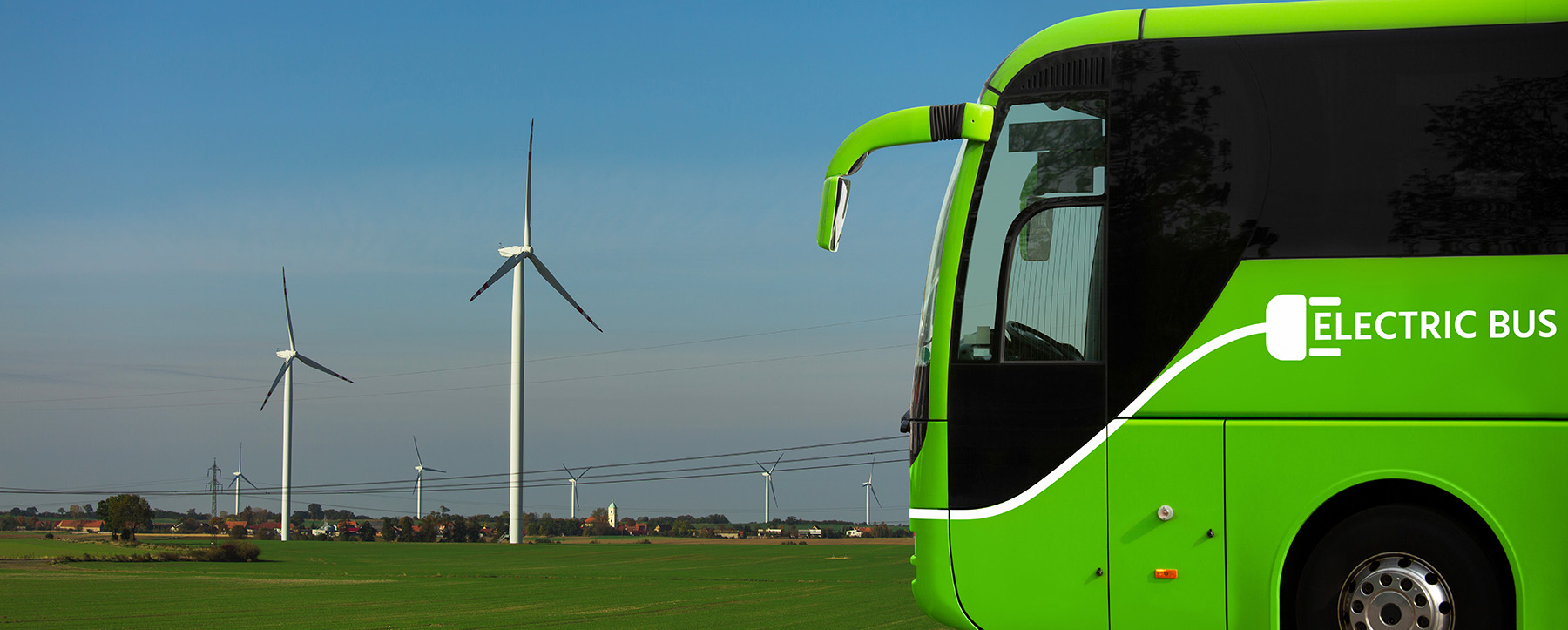
The nation should also aim for 50% of all new vehicle sales to be electric by 2030 and to develop a nationwide electric vehicle charging network. In existing buildings, at least 20% of all fossil-fuel-powered furnaces should be replaced with electric heat pumps while all new construction should be electrically heated “except in the coldest climate zones,” the summary document states. By 2030, the nation also needs to reduce total energy usage in new buildings by 50%; energy usage in existing buildings should be reduced by 30%, the report concludes. At the same time, the nation’s electrical grid should aim to increase transmission capacity by 40% to “better distribute high quality and low-cost wind and solar power” across the country.
America’s energy grid needs more storage capacity to accommodate renewable energy sources, Hendrickson says. This storage can range from traditional methods such as pumped storage to new, utility-scale battery systems that provide hundreds of megawatts of capacity. Storage is also critical to carbon sequestration plans, which involve capturing the emissions from traditional power plants — such as natural-gas-powered facilities — and then putting the greenhouse gases someplace where they will not get into the atmosphere, such as in vacant underground spaces, or chemically converting them into rocks, Hendrickson says.
These measures are achievable, Hendrickson adds, although the economics can be challenging.
Building better
Decarbonizing the energy grid is just the first of three key goals aimed at helping cities become net zero in terms of greenhouse gas emissions, says Erin McConahey, P.E., LEED BD+C, a principal in the Los Angeles office of the built environment consulting firm Arup. The second net-zero goal involves promoting greater energy efficiency within individual buildings to ensure that there will be sufficient capacity for the new electrical demands presented by the third key goal.
That goal involves the conversion of all appliances or systems that currently use oil, natural gas, or other fossil fuels — from stoves to water heaters — to run on electricity, McConahey says. Overall greater energy efficiency will also help ensure capacity for the expanded charging of electric vehicles, she adds.
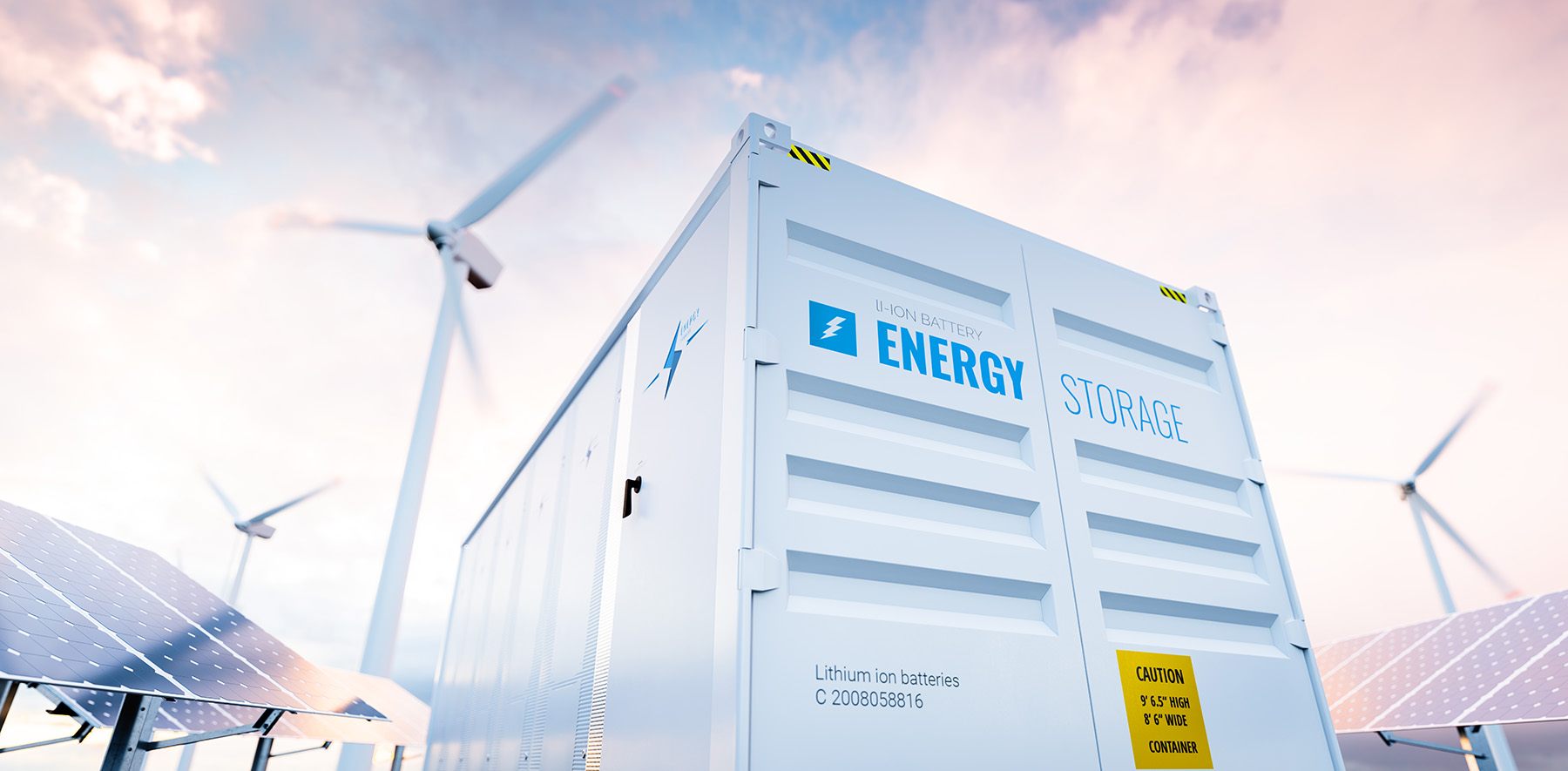
Arup worked with the city of Boston on the building sector portion of the Carbon Free Boston report, which examined more than 86,000 structures, ranging from office buildings to warehouses to schools. Working with data from Boston’s utilities, Arup calibrated information about individual building types and citywide energy performance. The team also considered future growth “to model scenarios to determine the combination of strategies capable of delivering the most significant (emissions) reductions,” according to the firm’s website on the project.
Many decarbonization efforts for buildings can be timed for the eventual natural replacement of fossil-fuel-based mechanical systems once they reach the end of their useful lives, rather than requiring building owners to rip out and replace carbon-intensive systems immediately, notes Pallavi Mantha, LEED AP, WELL AP, an associate in Arup’s New York City office.
But the electrification of mechanical systems in buildings does present various challenges. Electrically powered heat pumps, for example, can be far more efficient than traditional, fossil-fuel-powered boilers. And they are designed to both heat and cool buildings as a single system, instead of the two separate systems often used in traditional mechanical systems. But certain types of heat pumps generally need to interact with outside air and thus work best when located on rooftops.
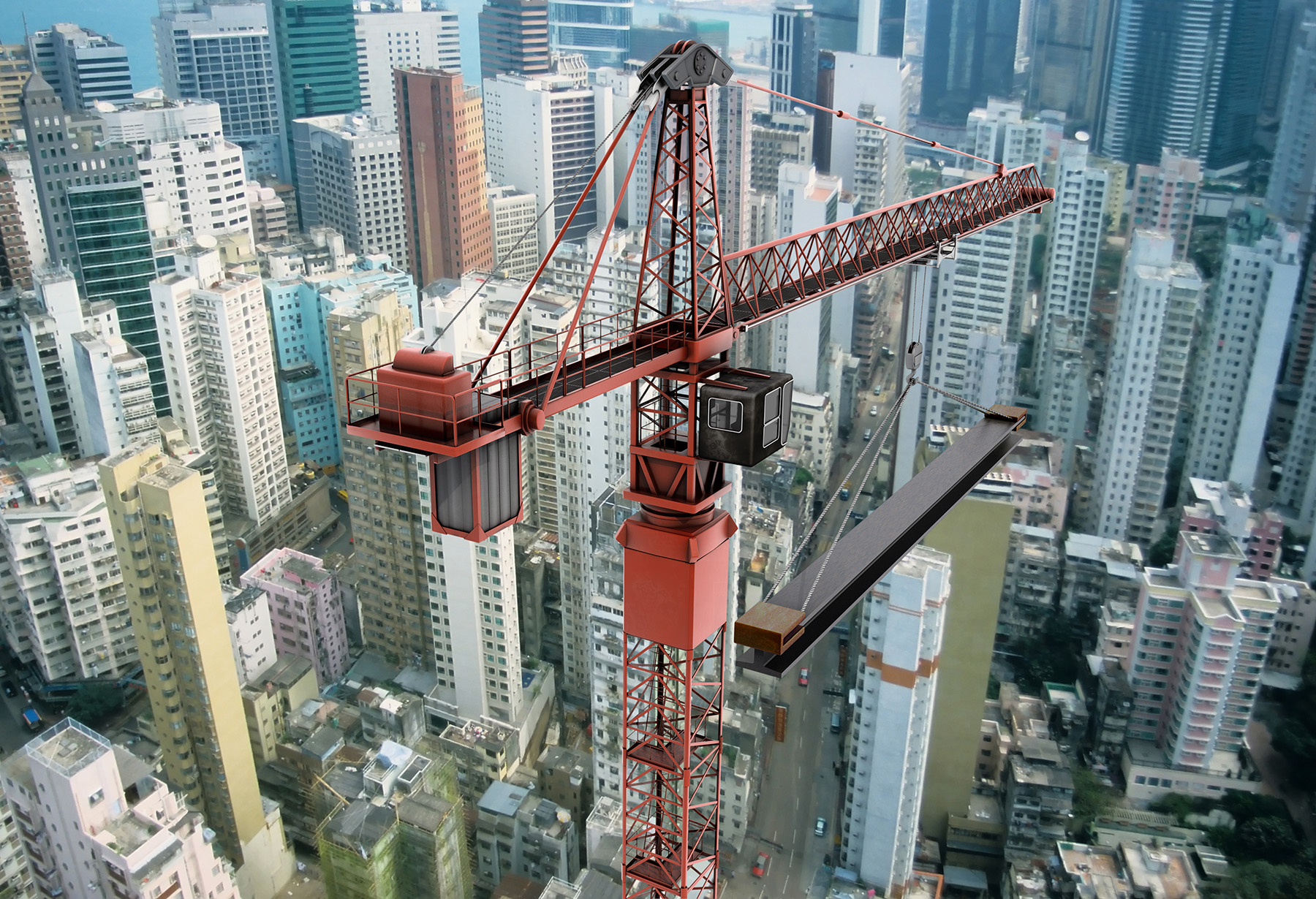
Plus, heat pumps require networks of piping for refrigerants — pipes that are somewhat limited by distance, says Mantha. So structural engineers must determine if the interior and roof of a particular building have the space and capacity to support the additional equipment, Mantha says.
The engineering firm Thornton Tomasetti worked with the city of Boston and the Boston-based Placetailor architecture firm on zero-emissions buildings guidelines that were aimed primarily at helping architects design energy-efficient affordable housing projects, notes Alejandra Menchaca, Ph.D., LEED AP, WELL AP, a vice president in the sustainability practice in Thornton Tomasetti’s Boston office. The engineering firm ran parametric analyses on the four or five most typical typologies of Boston’s affordable housing buildings, which tend to be between three and six stories tall, Menchaca says.
Thornton Tomasetti’s team ran thousands of computer-based simulations considering all possible variations of insulation, window styles, and other factors affecting energy performance, Menchaca explains.
The firm also worked with Boston to consider decarbonizing other public buildings, training city employees on how to better design net-zero buildings. “A lot of the effort should be made upfront,” Menchaca says. “Most of the analysis needs to be done in the study (and) schematic design phases, and the entire team needs to be involved.”
McConahey stresses the need for structural and other civil engineers to conduct whole life carbon assessments from a material basis. “We’re trying to encourage people to use life cycle assessments as a metric, the same as we’d use material cost as a metric, the same as material optimization is a metric,” she explains. “We’d just add an extra design parameter when you compare options at the beginning of a project to make clear to the owners what their carbon emissions exposure might be.”
Likewise, engineers should make environmental product declarations a bidding requirement for contractors to reveal what the likely emissions will be for materials, and clients can work to set emissions targets on projects, McConahey recommends.
Embodied issues
To move toward net-zero buildings, the United States needs to “significantly reduce the embodied carbon of buildings constructed during the next 10 years,” states a 2020 report, Embodied Carbon: A Clearer View of Carbon Emissions, which was produced by the engineering firm Walter P Moore. That can be challenging because many emissions reduction plans focus on the elimination of the carbon generated by operational aspects of buildings, but the embodied carbon in the structures has historically been overlooked, says Dirk Kestner, P.E., S.E., LEED AP BD+C, ENV SP, M.ASCE, a principal and the director of sustainable design in the Austin, Texas, office of Walter P Moore.
Fortunately, says Kestner, who helped write the Embodied Carbon report, there is a growing awareness that embodied carbon can be measured and compared from building to building. This information is vital for designing new structures and in deciding between various scenarios of retrofitting or repairing existing buildings, Kestner notes.
For structural engineers, it’s important to understand that the materials selected, the overall design, and even the location of a building can have a great impact on the structure’s embodied carbon. The choice of steel or concrete framing versus timber framing, for example, is key because the production of steel and concrete represents roughly 13-14% of global greenhouse gas emissions, while timber can actually store carbon, provided that it is obtained through sustainable forestry practices, says Patrick Kenny, P.E., a senior associate in Thornton Tomasetti’s Boston office. Kenny is part of the team leading the firm’s efforts to develop low-carbon specifications and embodied carbon structural designs.
Steel is often less carbon-intensive than concrete. That’s because concrete includes cement as a binding element and the chemical reaction needed to produce cement releases carbon dioxide as a byproduct, Kenny says. But structural engineers can influence the embodied carbon in concrete by specifying the use of lower-strength concretes — which require less cement — or cement replacement materials, such as slag or fly ash, in the concrete, he explains.
And while engineers cannot control the composition of steel the same way they can specify a concrete mix, they can select steel that was produced in an electric arc furnace — which generally uses recycled steel and can be powered by renewable electricity — rather than steel that was processed from iron ore in a basic oxygen furnace that burns coal, Kenny says.
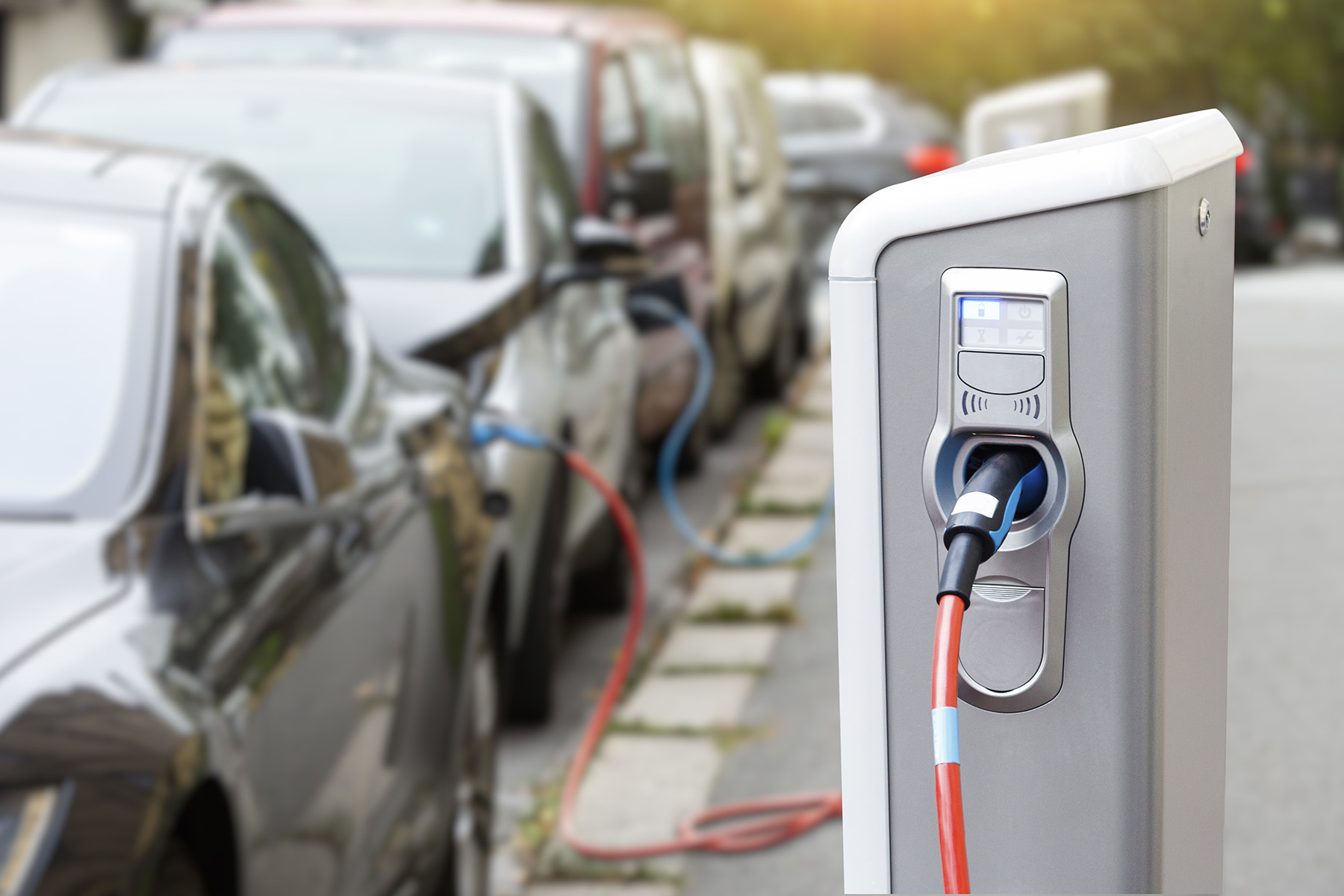
Prior to his current focus on reducing carbon, Kenny adds that he never thought much about the sources of the materials used in his projects or how they were produced. “But when you start looking into the carbon content, the carbon footprint, the embodied carbon, you must learn all about the supply chains,” Kenny says. “And that has been one of the most interesting parts about studying this (carbon) problem.”
In addition to the types of materials selected, the amounts used will also affect the embodied carbon — which is directly controlled by the architectural and structural design. “While column transfers, long spans, and offsets are an inevitable part of some designs, they all create a more complex load path and require more material,” Kestner writes in the Embodied Carbon report. This doesn’t mean that innovative designs should be avoided but rather that materials should be used in the most efficient manner possible. “More geometrically complex optimizations can be achieved through parametric analysis and organic optimization algorithms that allow teams to quickly assess multiple structural solutions,” the report explains.
Even the soil conditions at any particular site can have a major impact on the embodied carbon of a building because of the depth of piles or thickness of mats required. “The same office building in one part of a city might have a different embodied carbon based on the need for the amount of material that’s never seen because it’s used for the foundations,” Kestner explains.
He writes: “We must also consider how our design choices today may influence the ability of a building to be deconstructed in the future. We must transition our design thinking from a linear approach, where the end goal is the building, to a circular approach where buildings are thought of as material banks for the future. This will enable future design teams to more easily use salvaged materials.”
Driving down emissions
On the transportation side, decarbonization involves switching cars, trucks, and other vehicles from internal combustion engines to electrically powered propulsion that runs on batteries or fuel cells, notes Hendrickson. Improved efficiency in transportation is also critical and can be achieved in several ways that will help reduce emissions, such as by the use of connected and autonomous vehicles together with adaptive traffic signal systems to reduce traffic congestion and improve traffic flow overall, Hendrickson notes.
The use of reclaimed asphalt pavement can reduce lifetime greenhouse gas emissions in transportation infrastructure by as much as 65%, while shared rides, walking or bicycling, and even improved route planning for delivery vehicles can also help reduce emissions until the transportation system is electrified, Hendrickson wrote in an editorial, “Deep Decarbonization and Transportation Engineering,” which appeared in the July 2022 issue of ASCE’s Journal of Transportation Engineering, Part A: Systems. Hendrickson is the journal’s editor in chief and wrote the editorial with Laurence Rilett, Ph.D., P.E., F.ASCE, the director of Auburn University’s Transportation Research Institute.
In many ways, though, the conversion to electric vehicles “need not change” the existing roadway and other transportation infrastructure all that extensively, Hendrickson and Rilett wrote. Charging stations will have to be near roads, along with the necessary power transmission and distribution infrastructure, they noted. And some charging systems might be embedded directly in the road surfaces.
More significant, perhaps, will be the effects on transportation infrastructure caused by climate change and the need for transportation engineers to address those challenges — which are likely to occur whether the deep decarbonization of the transportation system happens or not, Hendrickson and Rilett pointed out.
Sea level rise, extreme weather, wildfires, mudslides, and other climate-related damages are already creating problems for transportation infrastructure operators, making infrastructure resiliency “increasingly important over the next decades,” Hendrickson and Rilett concluded.
Emissions and equity
There are numerous equity issues associated with decarbonization, Hendrickson adds, pointing out that much of the charging infrastructure for electric vehicles relies on people having access to dedicated parking spaces or garages — advantages that are not available to everyone. “So we’re going to need some sort of shared infrastructure for charging, both in residential areas and at (businesses) and for people making long trips,” Hendrickson says. Also, while electric vehicles have lower operating costs than conventional vehicles, they do have higher initial costs right now, which may be a hurdle for buyers, he adds.
The decisions about where such charging infrastructure is located should include the communities involved rather than being imposed from the top down, Hendrickson says. “In any sort of transition (from fossil fuels), there will be some winners and some losers,” he points out. As Hendrickson and Rilett wrote in the editorial: “It is important that deep decarbonization policies do not inequitably burden communities that have been historically disadvantaged by past transportation policy.”
Equity is also a concern when planning the electrification of mechanical services in buildings, notes Mantha. People want to know “what happens when the power goes out?” she explains. That is why Arup is working with the New York State Energy Research and Development Authority to consider what moving from fossil fuels to an all-electric future will mean for single-family homeowners and tenants in disadvantaged communities. “These are the communities on the fringes and on the front lines of climate change impacts — and also the most vulnerable,” Mantha says.
Moreover, such properties often do not have high values, “so how much investment will be available in terms of the urgency of backup power you might need? And for how long?” Mantha asks. “These are the kinds of questions we are navigating right now.”
That is why civil engineers need to tie together climate change, vulnerabilities, hazard exposure, and decarbonization at the same time, Mantha says.
Robert L. Reid is the senior editor and features manager of Civil Engineering.
This article first appeared in the September/October issue of Civil Engineering as "Engineering for Net Zero."
The Structural Engineering Institute of ASCE supports the vision of SE 2050: Committing to Net Zero, with its mission to support the SE 2050 Challenge and transform the practice of structural engineering in a way that is holistic, firm-wide, project-based, and data-driven. By prioritizing reduction of embodied carbon, through the use of less and/or less impactful structural materials, participating firms can more easily work toward net-zero embodied carbon structural systems by 2050.