Design of Resilient, Engineered, Autonomous, and Multifunctional (DREAM) structures
Mariantonieta Gutierrez Soto, Ph.D
Core competencies
- Design optimization
- Evolutionary game theory
- Advanced hazard mitigation
- Vibration control
- Soft computing
- Structural engineering
Problems
Multiple natural hazards pose a significant threat to critical infrastructure in many parts of the world that directly affect people's lives. The protection of the built environment from natural hazards is a critical and complex issue facing civil engineers. With the increased interest in smart cities, there is a need for an intelligent built environment. The latest innovation to fight natural hazards is the development of smart structures. The goal is to design a new generation of smart structures equipped with sensors and control devices that can react in real-time during multiple hazards. Structural control methods have been the subject of significant research in the past 20 years, yet it still faces limitations to enable a wider adoption by the ASCE community.
Approach
The rationale to embrace a biomimetic built environment during extreme events has been articulated through the need to understand the tradeoffs among different disaster mitigation strategies that provide artificial intelligence characteristics to the structure needed to combat the external forces from extreme natural hazard events. Our research group develops data-driven methodologies that lie in the intersection of artificial intelligence, vibration control, evolutionary game theory, biomimicry, machine learning, and neural dynamic modeling.
Artificial Intelligence
Artificial intelligence (AI) aims to simulate human intelligence processes (input information, learning, knowledge, making meaning, reasoning, conclusions, self-reflection, and self-correction, etc.) by machines. In AI, an agent is an autonomous abstract or software entity that observes through sensors and acts upon an environment in an adaptive or intelligent manner. In agent-based modeling, the agents are identified, and their behavior is defined. We can model what each agent does and also model what the agents do together. Agent-based modeling is particularly appealing for large-scale problems because agents do not require that all the agents interact directly, nor need to have complete information about the system to act. Modeling for artificial agents is divided into simple reflex, goal-based, and learning agents. The complexity exists in modeling the interaction through the sensory systems and how they act upon the environment. Modeling how agents simplify the complexity to predict and function is a current challenge in agent-based modeling. Our research group aims to model the built environment using AI.
Structural Vibration Control
Most structural control methods have a centralized control system: one central controller makes control decisions using the data collected from sensors installed in the structure and dispatches them to control devices. In such a system, centralized control represents a single point of potential failure. To overcome this shortcoming, a decentralized control system replaces the centralized control system with several local control systems. In decentralized control, computations are performed in parallel in a distributed computational environment. A concept developed in AI, an agent is an autonomous entity that observes through sensors and acts upon an environment in an adaptive manner to achieve defined goals. Agents can enhance the structural control system's robustness where nearby agents can compensate for an agent's malfunctions.
Game Theory
While game theory has traditionally been studied in political, economics, and social science, the mathematical modeling of cooperation and competition is useful in modeling a machine's brain. Expressly, the control agent's brain is set up with strategies, which enable the computer to exhibit rational behavior. Game theory has been used in conjunction with AI for autonomous drones and robotic organizational tasks. Recently, the evolutionary game theory concept of replicator dynamics started immersing in engineering. Replicator dynamics models how natural selection affects the population according to their habitat in an environment based on a measured fitness function or pay-off. The number of individuals in each habitat varies due to the interaction and comparison with the average fitness of the total population.
Findings
Our research group recently developed novel data-driven methodologies that integrate artificial intelligence, game theory, and decentralized control. The created multi-agent replicator controller mitigates the vibrations of structures subjected to dynamic loading. The procedure takes a large building structure and decomposes it into substructures, with each substructure having its controller and set of control devices, which define the agents. The agents communicate with each other through sensors and make decisions using replicator dynamics (a concept from evolutionary game theory) to achieve performance objectives while optimally allocating control device forces requiring energy consumption. Each player (control device) competes for available resources to achieve a defined goal: keep the building safe (e.g., reducing inter-story drift, floor acceleration, etc.) with a limited energy supply. The novel control methodology was evaluated using numerical simulations of building structures subjected to earthquakes and corresponding conventional control algorithms. The method was augmented by studying a patented neural dynamics model. We studied the proposed method effectiveness in reducing the vibrations of low- and high-rise buildings, smart base-isolated structures, and highway bridge structures. Our research team studies the control method's robustness with uncertain structural parameters and performance under selective technology failure (sensor/actuator malfunction) using machine learning techniques.
Our research group is also integrating traditionally separate fields: structural control and structural health monitoring. Structural health monitoring is to detect damage in the structure. During extreme events, the control system can use the health monitoring system's information and adapt to these changes in the structure, especially for multi-hazard resiliency. We can model a structure as an autonomous system with a self-sensing and monitoring and self-diagnosis and prognosis with intelligence leading to self-healing and repair. Robotics and aerospace structures strategies for vibration mitigation integrate these abilities. We examine these innovative solutions to design a resilient and sustainable built environment.
Cyber-Physical Testing
The adoption of smart damping systems requires the appropriate transfer of technology and the demonstration of its performance. Hybrid simulation is a cyber-physical technique that combines the experimental-analytic simulation of structures to study dynamic loading response. It is an attractive alternative for structural testing because it allows the representation of a large structure into physical and numerical sub-structures where elements of interest that are difficult to model can be the physical sub-structure. For example, a rate-dependent damping device is tested in the lab, while the numerical model consists of a tall structure. Our research team has studied control methodologies to handle time delays, actuator delays, and other related challenges to improve the accuracy of these cyber-physical experimentation techniques. The goal is bridging the gap in the broader adoption of these innovative techniques by demonstrating the potential contribution to society.
Aerodynamic mitigation through morphing building envelope
The building envelop plays a crucial role in sustainable energy and multi-hazard mitigation. During normal operation conditions, the effective control of a climate adaptive building envelop can enhance energy usage. During extreme events, loss of the components and cladding during these high wind events usually results in the entire building's loss. While the structural elements are still sound, the cost to repair damage from water intrusion results in large amounts of construction waste. We studied an origami-inspired smart building envelope that morphs in real-time to redistribute loading during wind conditions so that in a hurricane or high wind event, the building's components and cladding can autonomously redirect the loading path. High-fidelity computational fluid dynamics analysis results show the proposed morphing facade mitigating the vibrations for up to 42%. We have also investigated the proposed origami-shape configurations using additive manufacturing and study the aerodynamic effects through wind tunnel testing.
Impact
Our research vision is to enable the Design of Resilient, Engineered, Autonomous, and Multifunctional (DREAM) structures to improve communities' resilience of the built environment to hazards. Current smart structures do not explicitly consider integrating artificial intelligence, game theory, distributed control, system identification, and damage detection, nor do they quantify how these systems truly affect overall community resilience in the long term. Our research group contributions demonstrate how fundamental theory related to structural engineering combined with multi-disciplinary methods advances the built environment's resilience and sustainability. Our research group executes foundational knowledge about multi-hazard mitigation for smart cities building at the convergence of game theory, artificial intelligence, and smart structures. Our research developing data-driven modeling approaches helps mitigate the impact of multiple natural hazards, capturing complex behavior of building systems, and quantifying life-cycle benefits and resilience impacts of the proposed unique methodologies. Our contributions result in novel methodologies that will ultimately advance scientific understanding across various fields, including computer science, decision-making sciences, cyber-physical systems, control engineering, and structural engineering.
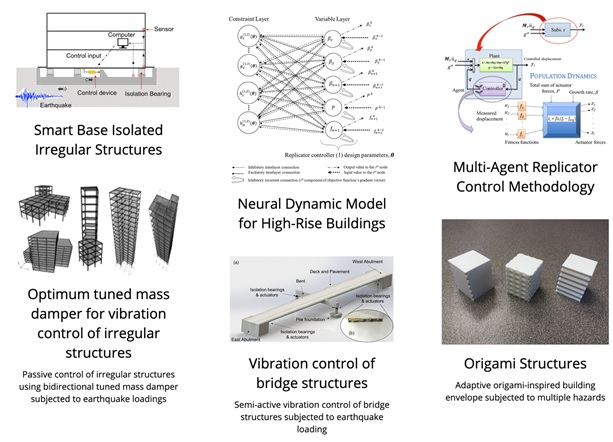
Current Researchers
(listed in alphabetical order per role)
- Mariantonieta Gutierrez Soto (Faculty)
- Prethesha Alagusundaramoorthy (Ph.D. student)
- Sajad Javadinasab Hormozabad (Ph.D. student)
- Laura Letellier (Ph.D. student)
- Alejandro Palacio Betancur (Ph.D. student)
- Phillip Thompson (Ph.D. student)
- Christopher Wheatley (Undergraduate student)
- Gabriela Gonzalez (High school student)
Alumni
(listed in alphabetical order per role)
- Amanda Bellamy (M.S. Civil Eng. and M.A. Architecture)
- Amanda Melendez (M.S. Civil Eng.)
- Roberto Barajas (B.S. Civil Eng.)
- Brandon Enbody (B.S. Civil Eng.)
- Daniel Gallutia (B.S. Civil Eng.)
- Caylee Marshall (B.S. Chemical Eng.)
- Alex Nguyen (B.S. Computer Eng.)
- Pablo Velasquez (B.S. Electrical Eng.)
- Chris Addington (Undergraduate student)
- Morgan Baumann (Undergraduate student)
- Kevin Tran (Undergraduate student)
- Nicolas Vargas (Undergraduate student)