Macroelements for Soil-Pile-Structure Interaction Analyses including Liquefaction
Georgia Institute of Technology
Problems
Pile foundations are by and large used for structures, piers and platforms on loose or soft soils, prone to liquefaction and lateral spreading during strong seismic events. The most widely employed approach for dynamic soil‐structure interaction analyses of piles in liquefiable soils is the Beam on Nonlinear Winkler Foundation (BNWF), according to which, the stiffness of pile springs (p‐y elements) derived for stiff, non‐ liquefiable soils is uniformly scaled via empirical factors that account for the reduction of soil resistance to seismic loading due to the development of excess pore water pressures. As a result, the hysteretic response of piles in loose saturated soils predicted via BNWF models is a scaled replica of the strain‐softening response of piles in stiff soils. Nonetheless, field and laboratory experimental data clearly show that the macroscopic response of piles in liquefiable soils is strain‐hardening, and that rate‐dependent phenomena due to radiation damping and seepage that cannot be simulated by the widespread BNWF models dominate the pile response.
Approach
Bridging the gap between widely employed empirical models and computationally expensive finite element simulations, we developed a macroelement for soil‐ structure interaction analyses of piles in liquefiable soils, which captures fundamental mechanisms of saturated granular soil response to dynamic loading such as dilation and seepage, while retaining the efficiency of substructure approaches for the analysis of seismic soil‐structure interaction problems. The macroelement components were developed using three‐dimensional fully coupled finite element (FE) analyses due to the limited number of available small and large‐scale experimental data. The FE simulations used for the parametric investigation and calibration of the macroelement were first validated using field data of blast‐induced liquefaction experiments.
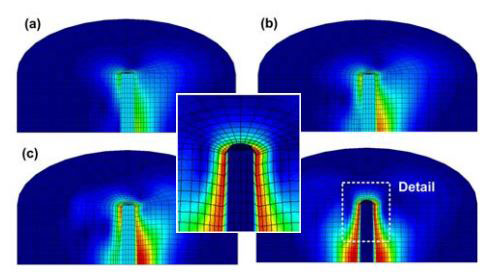
Findings
Using dimensional analysis, we identified the hydraulic conductivity, loading rate of dynamic loading, dilation angle and number of cycles to liquefaction as the soil properties dominating the response, and formulated accordingly the macroelement as a function of these physical parameters. Next, field data and numerical simulations highlighted the formation of a zone in the vicinity of the pile (near‐field) where the pore‐pressures differ substantially from the far‐field due to dilation effects and negative pressures in the shadow side of the pile (i.e. opposite to the direction of loading). By means of a pore‐pressure component, our macroelement can simulate these so‐called pile‐ induced liquefaction phenomena, as well as subsequent drainage of the pore‐fluid toward the far‐field. Results compared with field and laboratory experimental data were found to be in excellent agreement. The macroelement has been integrated in the open source finite element platform OPENSEES, and has been used in an NSF‐funded project of seismic hazard assessment and mitigation of liquefaction in port waterfront structures.
Impact
The pile macroelement advances the state‐of‐the‐art by efficiently providing realistic predictions of pile displacement in liquefiable soils. It allows the investigation of effectiveness of mitigation measures against liquefaction such as vertical drains. Bridge foundations, waterfront structures and highrise buildings are a few examples of critical infrastructure for which the macroelement will enable credible and cost‐effective design solutions. Furthermore, we are currently using the pile macroelement as a building block for novel soil‐ structure interaction mechanical models of fixed offshore wind turbine foundations. Offshore wind‐ generated electricity is foreseen as a major contributor to the US energy supply. The high cost of offshore wind projects, however, is one of the most important barriers that commercialization of offshore wind energy faces, and a significant contributor is the cost of wind turbine foundations, approximately 25% of the total cost of a wind farm. To date, no standards exist in the US for the design of foundations in the offshore wind industry. We envision that the macroelements for offshore wind turbine foundations will lead to cost‐effective design solutions in a renewable energy market with enormous national potential. Our pending European partnerships will provide valuable performance data and operational lessons for calibration and validation of our models.
Core competencies
- Fully-coupled numerical simulations of soil structure interaction problems
- Nonlinear site response analyses in seismology and engineering
- Topographic amplification of seismic ground motion in 2D and 3D
- Dynamic slope stability modeling
Current research team members
- Dominic Assimaki (PI)
- Seokho Jeong (Ph.D. Candidate)
- Kami Mohammadi (Ph.D. Candidate)
Recent graduates and co‐workers
- Varun Varun (Ph.D. Candidate)
- Wei Li (Ph.D. Candidate)
Current research collaborations
- Loukas Kallivokas in the Department of Civil Engineering at the University of Texas at Austin
- Adrian Rodriguez‐Marek in the Department of Civil Engineering at Virginia Tech